In this first post I will try and describe the project I will be mainly undertaking during my time in
Leuven. It has to do with accretion, and specifically the similarities observed within different accreting systems. Accretion is a ubiquitous phenomenon in the Universe, and is responsible for the growth of objects of all masses, from stellar-like objects to super-massive black holes at the centre of galaxies.
My project will specifically focus on a subset of accreting close interacting binary stars called
cataclysmic variables. These systems usually consist of two stellar mass objects orbiting each other, but one of them is not actually a star but a
white dwarf. The white dwarf is more massive that the star (although not by much on astronomical scales) however it's radius is much smaller. To make the point, one can imagine a white dwarf as having the same mass of our Sun, but all squished in a ball whose radius is that of planet Earth! The interesting and fascinating thing about cataclysmic variables (and indeed all close interacting binaries) is that the central compact object (a white dwarf in the case of cataclysmic variables) and the companion star are so close to each other that the gravitational potential of the compact object strips matter from the envelope of the secondary star.
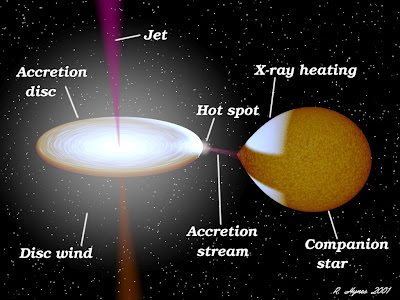 |
Schematic view of a compact interacting binary. Credit: R. Hynes |
This stripped matter is then on it's way to the white dwarf, and forms a disk in order to conserve angular momentum. This disk is very dynamic, and for material to get closer to the central white dwarf, angular momentum must be lost through interactions with surrounding material. For example,
viscous interactions is a mechanism by which angular momentum is lost (but defiantly not the only one!), and matter can gradually get closer and closer to the white dwarf, until it can settle on it's surface and increase it's mass by a bit. The issue however is that the laws that govern the physics within
accretion disks are very hard to grasp, since within these disks many effects come to play, like viscosity, magnetic fields, radiation, and in some cases also relativity. Models which seek to reproduce the observed behaviour within these disks sometimes get it right, but most times are wrong! If we were to understand fully how these disks behave and how they relate to the systems which produce them we would be able to also understand better how the systems evolve in the first place, and what their fate will be. This in turn can have direct implications to how galaxies evolve, since white dwarfs are the end-state of most stars in the Universe and most stars are also found in binaries. Supernova Ia are also the result of a white dwarf gaining so much mass (presumably by accretion) that it surpasses the
Chandrasekhar limit, resulting in a supernova explosion which can be seen throughout the Universe and can be used to measure it's size!
My project will relate some of the observed phenomena within the disks of cataclysmic variables to their more massive counterparts: X-ray binaries and Active Galactic Nuclei.
X-ray binaries are also close interacting binary systems, however the accretor in these cases is not a white dwarf but a more massive
black hole or
neutron star. On top of being more massive, black holes and neutron stars are also much smaller! In fact, if a white dwarf has the mass of the sun squished into the radius of the Earth, a neutron star is even more massive, and is squished into the size of a city! Black holes take it even a step further and are so dense that we don't even refer to it's surface any more but to the event horizon: the point where nothing, not even light, can escape from it's gravitational pull. For comparison, a stellar-mass black hole will have it's event horizon at just a few kilometres!
Active Galactic Nuclei on the other hand are not binary systems, but accreting super-massive black holes (with masses reaching a few million times the mass of the Sun), usually situated at the centre of Galaxies. Although at first all these systems might seem wildly different in both mass and size, they all have one common feature: an accretion disk! It is the similarities between these different sized disks which fuelled a lot of (what I think is) interesting research in the last few years: the fact that although these disks are seemingly different, there exist relations which, if scaled properly, allow us the see that they all behave in a very similar way. Cataclysmic variables offer a nice way to study these similarities since there are many more of these systems in the galaxy than there are X-ray binaries. If we can learn from the numerous cataclysmic variables and find similar behaviour to the X-ray binaries, then we can hope to get a step closer in understanding how all these different systems behave and relate to each other, including Active Galactic Nuclei!
Ciao!
Simo